Autocollimator: Precision Alignment Tool for Optical Systems
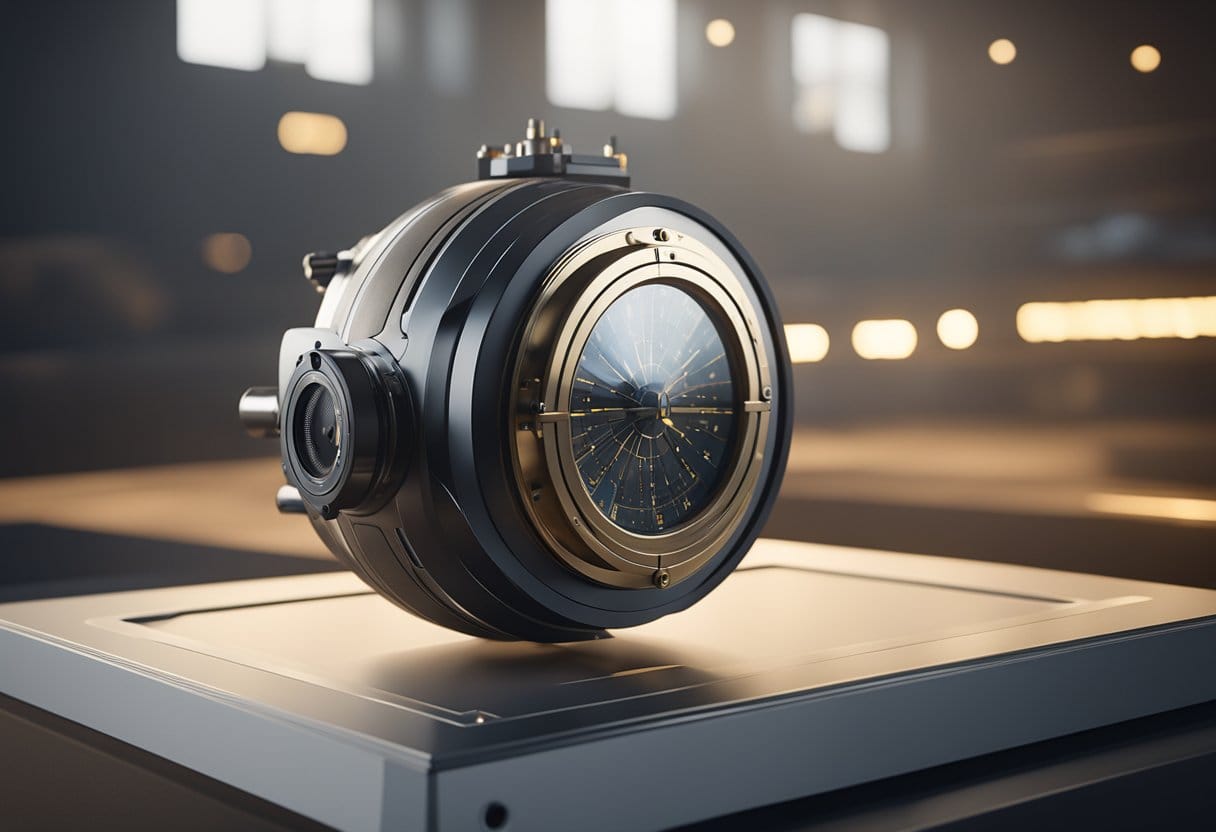
Precision and accuracy are paramount in many scientific and engineering fields. When it comes to measuring minute angular displacements, few tools are as effective as an autocollimator. An autocollimator is an optical instrument that uses a collimated light beam to measure angular deviations with remarkable sensitivity, typically in the range of fractions of an arcsecond.

Autocollimators work by projecting a beam of light onto a reflective surface and analyzing the returned beam. The angular displacement between the emitted and reflected beams provides crucial data for alignment and calibration in various industries. These devices find applications in fields such as optics, aerospace, and precision manufacturing.
The versatility of autocollimators is evident in their diverse applications. From aligning optical components to measuring flatness and straightness of machined parts, these instruments play a vital role in ensuring the highest standards of precision. As technology advances, electronic autocollimators have emerged, offering enhanced accuracy and digital data processing capabilities.
History of Autocollimators

Autocollimators have a rich history dating back to the early 19th century. The concept was first introduced by German physicist Carl Friedrich Gauss in 1821.
Gauss developed the initial idea while working on geodetic surveys. He aimed to create a device that could accurately measure small angular deviations.
In 1850, Julius Plucker, another German physicist, further refined the concept. He introduced the use of a reticle pattern to improve measurement precision.
The modern autocollimator design emerged in the early 20th century. It incorporated advancements in optics and precision engineering.
During World War II, autocollimators played a crucial role in military applications. They were used for aligning gun sights and other optical instruments.
In the 1960s, electronic autocollimators were developed. These devices used photodetectors instead of visual observation, increasing accuracy and speed.
Today, autocollimators are essential tools in various industries. They are used for high-precision alignment and angle measurement in manufacturing, aerospace, and scientific research.
Recent advancements include digital autocollimators with computer interfaces. These allow for automated measurements and data analysis, further enhancing their capabilities.
Principles of Operation

Autocollimators operate on fundamental optical principles to measure angular deviations with high precision. These instruments utilize collimated light beams and reflective surfaces to detect minute angular changes.
Optical System Fundamentals
The autocollimator's optical system relies on two key principles. First, it projects and refracts a parallel beam of light using a lens. Second, it detects changes in the direction of a reflected beam from a plane reflecting surface.
A monochromatic light source generates the initial beam. This beam passes through a beam deflector, which directs it towards a converging lens. The lens collimates the light, creating parallel rays.
The collimated beam exits the instrument and reflects off an external surface. Any angular deviation in this surface affects the beam's return path.
Alignment and Measurement Process
The alignment process begins with the emission of a collimated beam towards the target reflective surface. This surface can be a mirror, polished metal, or any flat reflective object under test.
The reflected beam re-enters the autocollimator through the same optical path. If the reflective surface is perfectly perpendicular to the incident beam, the return beam follows the exact path of the emitted beam.
Any angular misalignment of the reflective surface causes a deviation in the return beam's path. This deviation is proportional to the angular error of the surface.
The instrument's internal optics focus the return beam onto a detection system. This system precisely measures the beam's position relative to its original path.
Angle Deviation Detection
The heart of the autocollimator's detection system is a photodetector or an image sensor. This component captures the position of the focused return beam.
For small angular deviations, the displacement of the return beam on the detector is directly proportional to the angle. This relationship allows for precise angular measurements.
Modern autocollimators often use digital image processing techniques. These methods enhance measurement accuracy and enable real-time analysis of angular deviations.
The instrument's software interprets the detector data, converting beam displacements into angular measurements. It can display results in various units, such as arc-seconds or microradians.
Types of Autocollimators
Autocollimators come in several varieties, each designed for specific measurement needs and operating conditions. These instruments differ in their detection methods, light sources, and capabilities, offering varying degrees of precision and versatility.
Visual Autocollimators
Visual autocollimators rely on the human eye for detection and measurement. They project an illuminated reticle onto a reflective surface and use the reflected image for angular measurements. These devices typically offer a resolution of 3 to 5 arc-seconds.
Visual autocollimators are often preferred for their simplicity and durability. They don't require power sources, making them suitable for field use. However, measurement accuracy can vary between operators due to differences in visual acuity.
These instruments excel in alignment tasks, such as checking the parallelism of optical components. They can measure multiple surfaces simultaneously, enhancing efficiency in certain applications.
Electronic Autocollimators
Electronic autocollimators use photodetectors instead of the human eye to measure angular deviations. They offer higher precision and automation capabilities compared to visual models. These devices can achieve resolutions down to 0.1 arc-seconds.
Electronic autocollimators provide digital readouts, eliminating operator-dependent variations. They can interface with computers for data logging and analysis, making them ideal for quality control and research applications.
These instruments often feature built-in calibration and can maintain accuracy over longer working distances. Their ability to detect minute angular changes makes them valuable in high-precision manufacturing and scientific research.
Laser Autocollimators
Laser autocollimators utilize laser light sources for enhanced accuracy and extended measurement range. They combine the principles of autocollimation with laser interferometry to achieve exceptionally high precision.
These devices can measure angular deviations over distances up to 30 meters, surpassing the capabilities of traditional autocollimators. Laser autocollimators are particularly useful in large-scale alignment tasks and metrology applications.
Their narrow beam width allows for measurements of small targets or in confined spaces. Laser autocollimators often incorporate advanced features like automatic target recognition and real-time position tracking, enhancing their versatility in dynamic measurement scenarios.
Components and Design
An autocollimator consists of several key optical and electronic components that work together to enable precise angular measurements. Its design incorporates a light source, collimating optics, a beamsplitter, and detection systems to project and analyze reflected light beams.
Main Optical Components
The heart of an autocollimator's optical system is the collimating lens. This lens produces a parallel beam of light from the illumination source. A beamsplitter directs the collimated light towards the target mirror.
The target mirror reflects the beam back through the beamsplitter. Any angular deviation of the mirror causes a displacement of the returned beam. This displacement is measured to determine the mirror's angular position.
High-quality optical components are crucial for accuracy. Precision-ground lenses and flat mirrors with surface accuracies in the range of λ/10 or better are commonly used.
Eyepiece and Reticle
Visual autocollimators incorporate an eyepiece for direct observation. The eyepiece magnifies the returned image, allowing for precise alignment.
A reticle, typically a glass plate with etched crosshairs or measurement scales, is placed at the focal plane. This reticle serves as a reference point for measuring beam displacement.
Some advanced systems use digital displays instead of traditional eyepieces. These provide numerical readouts of angular measurements, enhancing precision and ease of use.
Detectors and Sensors
Modern autocollimators often employ electronic detectors for automated measurements. Common types include:
- Photodiode arrays
- CCD (Charge-Coupled Device) sensors
- CMOS (Complementary Metal-Oxide-Semiconductor) sensors
These detectors convert the position of the returned light beam into electrical signals. Signal processing algorithms then calculate the angular deviation with high precision.
Electronic autocollimators can achieve resolutions as fine as 0.01 arcseconds, surpassing the capabilities of visual systems.
Illumination Systems
The illumination system provides the light source for the autocollimator. LED (Light-Emitting Diode) sources are commonly used due to their long life and stability.
For specialized applications, laser sources may be employed. These provide extremely coherent and collimated light, enhancing measurement precision.
The intensity and wavelength of the light source are carefully chosen to optimize visibility and minimize measurement errors due to chromatic aberration.
Illumination systems often include adjustable brightness controls. This allows operators to optimize the light level for different target surfaces and ambient lighting conditions.
Applications of Autocollimators
Autocollimators play a crucial role in various industries and scientific fields. These precision instruments enable highly accurate angular measurements and alignments, making them indispensable for quality control, research, and development across multiple sectors.
Engineering and Machining
In engineering and machining, autocollimators are essential for ensuring precision and accuracy. They are used to verify angle standards and detect minute angular movements in machinery and components. Autocollimators help maintain tight tolerances in manufacturing processes, especially for high-precision parts.
These devices are particularly valuable in the production of machine tools, where angular alignment is critical. They assist in calibrating rotary tables, indexing heads, and other precision equipment. Autocollimators also play a key role in measuring the flatness and parallelism of surfaces, which is crucial for quality control in machining operations.
In the automotive industry, autocollimators are used to align components in engines and transmissions. They ensure that critical parts are positioned correctly, contributing to improved performance and longevity of vehicles.
Optical Component Testing
Autocollimators are indispensable in the optical industry for testing and aligning various components. They are used to check the face parallelism of optical windows and wedges, ensuring that light passes through these elements as intended.
In lens production, autocollimators help verify the curvature and alignment of lens surfaces. This is crucial for producing high-quality lenses for cameras, microscopes, and other optical instruments. The devices are also used to test mirrors, prisms, and other reflective surfaces for flatness and angular accuracy.
Autocollimators aid in the assembly and testing of complex optical systems like telescopes and spectrometers. They ensure that all components are precisely aligned, which is essential for optimal performance. In laser systems, autocollimators are used to align rod ends and verify beam collimation.
Astronomy and Telescopes
In astronomy, autocollimators are vital for the construction and maintenance of telescopes. They help align the primary and secondary mirrors in reflecting telescopes, ensuring that light is focused accurately onto the detector or eyepiece.
These instruments are used to calibrate telescope mounts and tracking systems. By providing precise angular measurements, autocollimators enable astronomers to accurately point telescopes at celestial objects and maintain their position during long exposure times.
In radio astronomy, autocollimators assist in aligning the large dish antennas used to detect radio waves from space. They ensure that these massive structures are positioned correctly to capture faint signals from distant cosmic sources.
Research and Development
Autocollimators find extensive use in various research and development settings. In physics laboratories, they are employed for precision alignment in experimental setups, such as laser interferometry and spectroscopy.
These devices are crucial in the development of new optical technologies. They help researchers test and refine prototypes of optical components and systems, ensuring that innovations meet the required specifications. Autocollimators are also used in the study of material properties, helping researchers measure minute deformations and stress-induced changes in samples.
In the aerospace industry, autocollimators play a role in developing and testing guidance systems and optical sensors for satellites and spacecraft. They ensure that these critical components are aligned with the highest degree of accuracy, which is essential for successful space missions.
Calibration and Maintenance
Proper calibration and maintenance are crucial for ensuring the accuracy and longevity of autocollimators. These processes involve routine procedures, preventive measures, and careful error management.
Routine Calibration Procedures
Autocollimators require regular calibration to maintain their precision. Electronic autocollimators used in comparisons often undergo calibration across their full range, typically ±1000 arcseconds in 10 arcsecond steps.
The calibration process may involve using a rotation mechanism to generate small angles. These angles are then measured by both the calibration standard and the autocollimator being tested.
Some facilities use Digital Small Angle Generators (DSAG) for calibration checks. Fixed test wedges can provide quick accuracy checks between full calibrations.
Preventive Maintenance
Regular maintenance helps prevent performance degradation and extends the life of autocollimators. This includes keeping optical components clean and free from dust or scratches.
Technicians should regularly inspect the autocollimator's housing for any signs of damage or misalignment. They must ensure all electrical connections are secure and free from corrosion.
Environmental controls are crucial. Autocollimators should be stored and operated in temperature-stable, low-humidity environments to prevent condensation on optical surfaces.
Proper handling during transport and setup is essential. Technicians should use designated carrying cases and follow manufacturer guidelines for installation and leveling.
Error and Uncertainty Management
Understanding and managing errors is critical for accurate autocollimator measurements. Systematic errors can arise from misalignment of the beam-limiting aperture relative to the optical axis.
Calibration standards themselves introduce uncertainties. Electronic levels, while offering advantages in size and cost, have limitations that must be considered in the calibration process.
To minimize errors, operators should ensure consistent alignment procedures and maintain stable environmental conditions during measurements. Regular comparison to primary angle standards, such as the Heidenhain WMT 220 angle comparator, helps quantify and reduce uncertainties.
Proper documentation of calibration results, including uncertainty budgets, is essential for traceability and long-term performance monitoring.
Advancements in Autocollimator Technology

Recent years have seen significant improvements in autocollimator technology. These advancements have expanded the capabilities and applications of these precision optical instruments.
One major development is the introduction of digital autocollimators. Unlike their visual counterparts, digital models use electronic sensors to detect and measure angular deviations with greater accuracy and speed.
Enhanced resolution is another key area of progress. Modern autocollimators can achieve remarkably precise measurements, often in the sub-arcsecond range. This increased precision enables more accurate alignment and calibration in various industries.
Autocollimators have also become more versatile. New technologies allow for direct measurements of incoming laser beams, opening up possibilities for inter-alignment between optics, mirrors, and lasers.
The integration of advanced software has further improved autocollimator functionality. These programs can process and analyze measurement data in real-time, providing instant feedback and facilitating more efficient alignment procedures.
Miniaturization efforts have resulted in more compact and portable autocollimator designs. This development has made these instruments more accessible for field work and on-site measurements.
Applications for autocollimators have expanded, now including performance characterization for AR/VR goggles, 3D LiDARs, and satellite alignment. This versatility has solidified the autocollimator's position as a crucial tool in modern optical metrology.
Selection and Procurement

When selecting an autocollimator, several key factors must be considered. The focal length of the instrument is crucial, as it determines the measuring range and accuracy. Longer focal lengths generally provide higher accuracy but with a smaller field of view.
The type of autocollimator is another important consideration. Electronic autocollimators offer advantages in terms of data acquisition and processing, while optical autocollimators may be more suitable for certain applications.
Resolution and accuracy specifications should be carefully evaluated based on the intended use. Some autocollimators can achieve accuracies as high as 0.2 arcseconds.
The specific application requirements must be taken into account. For instance, measuring vibrations or rapidly moving samples may necessitate a high-speed autocollimator with high measurement rates.
Budget constraints and available support from the manufacturer should also factor into the procurement decision. It's advisable to consult with reputable suppliers and compare specifications from different models before making a final selection.
Compatibility with existing equipment and software systems in the workplace is another crucial aspect to consider during the procurement process.
Safety and Handling Considerations

Autocollimators are precision optical instruments that require careful handling and maintenance. Proper safety measures are essential to protect both the equipment and users.
When working with autocollimators, always wear appropriate personal protective equipment (PPE) such as safety glasses to shield eyes from potential light reflections or beam scattering.
Handle the instrument with clean, dry hands or wear lint-free gloves to prevent contamination of optical surfaces. Avoid touching lenses, mirrors, or other optical components directly.
Store the autocollimator in a clean, dry environment when not in use. Use a protective case or cover to shield it from dust and moisture.
Temperature fluctuations can affect alignment accuracy, so maintain a stable ambient temperature during operation and calibration.
Regular cleaning is crucial. Use specialized optical cleaning solutions and lint-free wipes to gently remove dust or debris from optical surfaces.
Exercise caution when connecting electronic autocollimators to power sources. Ensure proper grounding and follow manufacturer guidelines for electrical safety.
When adjusting or aligning components, move slowly and deliberately to avoid accidental impacts or misalignments.
Train all users on proper handling techniques and safety protocols before allowing them to operate the autocollimator.