Laser Scanning Microscope: Advanced Imaging for Cellular Research
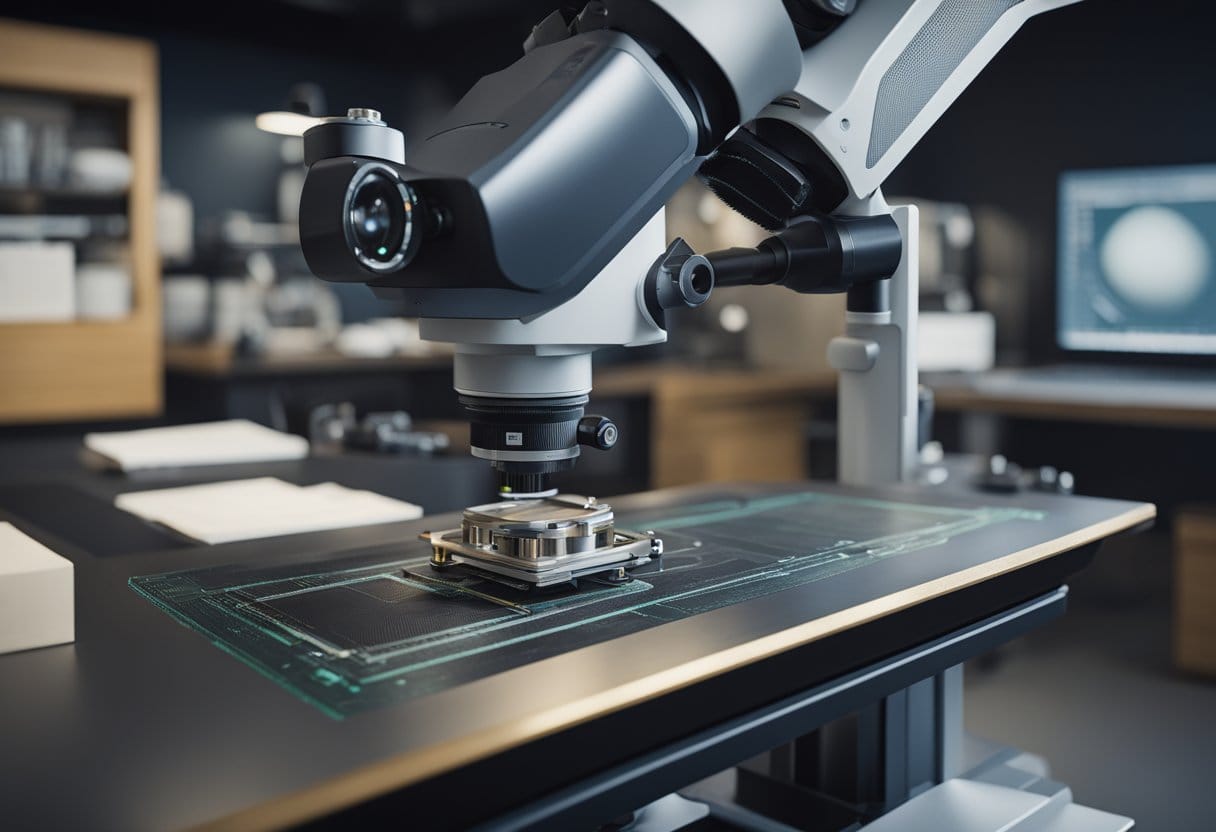
Laser scanning microscopes have revolutionized the field of cellular imaging. These advanced instruments use focused laser beams to scan specimens point by point, creating high-resolution, three-dimensional images of biological samples. Laser scanning microscopes enable scientists to study cells and tissues with unprecedented detail and clarity, offering significant advantages over traditional microscopy techniques.

One popular type of laser scanning microscope is the confocal laser scanning microscope (CLSM). This technology employs a spatial pinhole to block out-of-focus light, resulting in sharper images with improved optical resolution and contrast. CLSMs are widely used in various scientific disciplines, including biology, medicine, and materials science.
Recent advancements in laser scanning microscopy have led to the development of high-speed imaging capabilities. Modern systems can capture video-rate images of live physiological events, allowing researchers to observe dynamic cellular processes in real-time. These cutting-edge microscopes continue to push the boundaries of scientific discovery, providing invaluable insights into the microscopic world.
Principles of Laser Scanning Microscopy
Laser scanning microscopy relies on focused light beams, precise scanning mechanisms, and sophisticated detection systems. These elements work together to produce high-resolution images of microscopic samples.
Laser Fundamentals
Laser scanning microscopes utilize specific wavelengths of light to illuminate samples. The laser source emits a coherent beam of photons, typically in the visible or near-infrared spectrum. This beam is tightly focused to a diffraction-limited spot on the specimen.
The choice of laser wavelength affects resolution and penetration depth. Shorter wavelengths provide better resolution but may cause more photodamage. Longer wavelengths penetrate deeper into samples but sacrifice some resolving power.
Laser power is carefully controlled to balance image quality and sample preservation. Too much power can damage delicate biological specimens, while insufficient power results in weak signals.
Raster Scanning Technique
The focused laser beam is systematically moved across the sample in a raster pattern. This scanning is achieved using galvanometer mirrors or acousto-optic deflectors.
X and Y scanning mirrors direct the beam horizontally and vertically. The scanning speed can be adjusted to optimize image acquisition time and signal-to-noise ratio.
For 3D imaging, the focal plane is shifted along the Z-axis. This allows optical sectioning of thick samples, creating a stack of 2D images that can be reconstructed into a 3D volume.
Signal Detection and Processing
As the laser scans the sample, various signals are generated. These may include fluorescence, reflected light, or transmitted light. Sensitive detectors, such as photomultiplier tubes or avalanche photodiodes, capture these signals.
A pinhole aperture in the detection path blocks out-of-focus light, enhancing image contrast and resolution. This is the key principle of confocal microscopy.
The detected signals are digitized and processed to form an image. Advanced software allows for real-time visualization, noise reduction, and quantitative analysis of the acquired data.
Multi-channel detection enables simultaneous imaging of different fluorophores or contrast mechanisms, providing rich, multi-dimensional datasets.
Types of Laser Scanning Microscopes
Laser scanning microscopes utilize different techniques to generate high-resolution images of specimens. These advanced imaging systems employ various methods to excite fluorophores and capture detailed structural information at the cellular and subcellular levels.
Confocal Laser Scanning Microscopy
Confocal laser scanning microscopy (CLSM) is a widely used imaging technique that provides optical sectioning capabilities. It uses a focused laser beam to scan the specimen point by point, creating high-resolution 3D images.
The key components of a CLSM system include:
- Laser light source
- Scanning mirrors
- Objective lens
- Pinhole aperture
- Photomultiplier tube detector
Confocal laser scanning microscopes eliminate out-of-focus light by using a pinhole aperture, resulting in improved contrast and resolution compared to conventional microscopes. This technique allows for optical sectioning of thick specimens and the creation of 3D reconstructions.
CLSM is particularly useful for imaging fluorescently labeled samples in cell biology, neuroscience, and developmental biology research.
Two-Photon Excitation Microscopy
Two-photon excitation microscopy is a nonlinear optical imaging technique that offers several advantages over conventional confocal microscopy. It uses longer wavelength, near-infrared light to excite fluorophores.
Key features of two-photon microscopy include:
- Deeper tissue penetration
- Reduced photobleaching and phototoxicity
- Improved signal-to-noise ratio
This technique relies on the simultaneous absorption of two photons by a fluorophore, which only occurs at the focal point of the laser beam. This intrinsic optical sectioning capability eliminates the need for a pinhole aperture.
Two-photon microscopy is particularly valuable for imaging living tissues and organisms, as it causes less damage to samples and allows for longer observation periods.
Multiphoton Laser Scanning Microscopy
Multiphoton laser scanning microscopy extends the principles of two-photon excitation to include three-photon and higher-order nonlinear processes. This technique offers even greater imaging depths and reduced photodamage compared to two-photon microscopy.
Advantages of multiphoton microscopy include:
- Enhanced tissue penetration
- Improved spatial resolution
- Reduced background fluorescence
Multiphoton microscopy can excite a wider range of fluorophores and generate signals from endogenous molecules like collagen and NADH. This capability makes it valuable for studying tissue structure and metabolism in intact organs and live animals.
Applications of multiphoton microscopy span various fields, including neuroscience, cancer research, and regenerative medicine.
Components of a Laser Scanning Microscope

Laser scanning microscopes consist of several key components that work together to produce high-resolution images. These components include specialized optical sources, precise scanning systems, high-quality objective lenses, sensitive detectors and filters, and stable sample holders and stages.
Optical Sources
Laser scanning microscopes typically use lasers as their primary illumination source. Common laser types include argon-ion, helium-neon, and diode lasers. These lasers provide intense, monochromatic light at specific wavelengths.
Laser selection depends on the fluorophores or samples being imaged. Some systems utilize multiple lasers to excite different fluorescent markers simultaneously.
The laser beam is focused to a diffraction-limited spot, allowing for high-resolution imaging. Laser power can be adjusted to optimize signal-to-noise ratio while minimizing photobleaching and phototoxicity.
Scanning Systems
Scanning systems direct the laser beam across the sample in a precise pattern. Two main types are used:
- Galvanometer mirrors: These rapidly oscillating mirrors scan the beam in X and Y directions.
- Resonant scanners: These provide faster frame rates, ideal for live-cell imaging.
The scanning system works in tandem with the detection system to build up the image pixel by pixel. Fast scanning mirrors enable the acquisition of geometrically correct images.
Scan speed and pattern can be adjusted to balance image quality, acquisition speed, and sample preservation.
Objective Lenses
High-quality objective lenses are crucial for laser scanning microscopy. These lenses focus the laser beam onto the sample and collect emitted fluorescence.
Key characteristics include:
- High numerical aperture (NA) for improved resolution and light collection
- Specialized coatings to minimize aberrations and reflections
- Correction for chromatic and spherical aberrations
Objectives are often designed for specific imaging modalities, such as water-immersion lenses for live-cell imaging or oil-immersion lenses for fixed samples.
The choice of objective affects resolution, working distance, and field of view.
Detectors and Filters
Sensitive detectors capture the fluorescence emitted by the sample. Common types include:
- Photomultiplier tubes (PMTs): Offer high sensitivity and dynamic range
- Avalanche photodiodes (APDs): Provide improved quantum efficiency
Spectral detectors allow for simultaneous detection of multiple fluorophores.
Filters are essential for separating excitation and emission light:
- Dichroic mirrors reflect excitation light while transmitting emission
- Emission filters selectively pass fluorescence wavelengths
- Notch filters block scattered laser light
Confocal microscopes use pinholes to reject out-of-focus light, improving optical sectioning and resolution.
Sample Holders and Stages
Precise sample positioning and stability are crucial for high-quality imaging. Key components include:
- Motorized XY stages for automated scanning of large samples
- Z-focus drives for acquiring 3D image stacks
- Temperature-controlled chambers for live-cell imaging
- Vibration isolation tables to minimize environmental disturbances
Some systems incorporate piezoelectric stages for rapid, nanometer-scale positioning. This enables techniques like fast Z-stacks and photoactivation experiments.
Sample holders are designed for specific applications, such as multi-well plates for high-throughput screening or perfusion chambers for long-term live-cell imaging.
Applications and Capabilities
Laser scanning microscopes offer versatile imaging capabilities across diverse scientific and industrial fields. These advanced instruments enable high-resolution imaging, 3D reconstruction, and real-time analysis of various samples and materials.
Biological and Medical Research
Confocal laser scanning microscopy (CLSM) has revolutionized biological and medical research. It allows for detailed imaging of living cells, tissues, and organisms with exceptional clarity.
Researchers use CLSM to study cellular structures, protein interactions, and dynamic processes in real-time. This technique is particularly valuable in neuroscience for mapping neural networks and tracking cellular changes in brain tissue.
In dermatology, CLSM enables non-invasive examination of skin lesions, aiding in early detection of skin cancers. It also assists in evaluating the efficacy of topical treatments by visualizing drug penetration into skin layers.
Material Science and Engineering
Laser scanning microscopes play a crucial role in material science and engineering applications. They provide detailed surface analysis and 3D topography of various materials, from metals to polymers.
Engineers use these microscopes to inspect semiconductor wafers, detect manufacturing defects, and analyze material properties at the microscale. The high-resolution imaging capabilities allow for precise measurements of surface roughness and texture.
In the field of corrosion studies, laser scanning microscopes help visualize and quantify surface degradation over time. This information is vital for developing protective coatings and improving material durability.
Nanotechnology and Metrology
In nanotechnology, laser scanning microscopes are essential tools for characterizing and measuring nanoscale structures. They offer precise dimensional measurements and surface profiling of nanoparticles, thin films, and nanodevices.
These microscopes enable quality control in nanofabrication processes, ensuring the accuracy of nanoscale features in electronics and photonics. Researchers use them to study the properties of novel nanomaterials and optimize their synthesis methods.
Laser scanning microscopes also contribute to advances in metrology, providing traceable measurements for calibration standards and improving manufacturing precision across industries.
Fluorescence Imaging
Laser scanning microscopes excel in fluorescence imaging, offering high sensitivity and specificity for detecting fluorescent molecules. This capability is invaluable in life sciences research and diagnostic applications.
Scientists use fluorescence imaging to track specific proteins, study gene expression, and observe cellular processes in living specimens. Multi-color fluorescence imaging allows simultaneous visualization of different cellular components or molecules.
In medical diagnostics, fluorescence-based laser scanning microscopy aids in detecting pathogens, analyzing tissue samples, and identifying biomarkers for various diseases. This technique also supports drug discovery by enabling researchers to monitor drug interactions at the cellular level.
Sample Preparation and Handling
Proper sample preparation is crucial for obtaining high-quality images with a laser scanning microscope. Specimens must be carefully prepared to preserve their structure and fluorescence.
For fixed samples, clearing techniques can enhance imaging depth by removing light-scattering lipids. This allows for better visualization of internal structures in thick tissue samples.
Live cell imaging requires maintaining physiological conditions. Temperature-controlled stages and CO2 incubation chambers help keep cells viable during extended imaging sessions.
Fluorescent labeling is essential for most laser scanning microscopy applications. Common methods include:
- Immunofluorescence with antibodies
- Genetic encoding of fluorescent proteins
- Chemical dyes and probes
Sample mounting is another critical step. For fixed samples, antifade mounting media help preserve fluorescence. Live specimens often require specialized chambers or dishes.
When preparing adherent cells, protocols typically involve fixation, permeabilization, and immunolabeling. These steps must be optimized for each sample type and target molecule.
Proper handling of samples is crucial to avoid introducing artifacts. Minimize exposure to light and use appropriate storage conditions to maintain sample integrity before and during imaging sessions.
Data Analysis and Interpretation

Laser scanning microscopes generate vast amounts of complex data. Effective analysis and interpretation are crucial for extracting meaningful insights from these datasets. Advanced software tools and techniques enable researchers to perform quantitative analysis, reconstruct high-resolution images, and process data efficiently.
Quantitative Analysis
Laser scanning microscopes provide precise quantitative data on cellular structures and processes. Researchers can measure parameters such as fluorescence intensity, particle size, and colocalization of different molecules.
Image analysis software allows for automated counting and tracking of cells or subcellular components. This enables high-throughput studies of dynamic processes like cell migration or protein trafficking.
Statistical tools help scientists analyze large datasets, identifying significant trends and correlations. Machine learning algorithms can be applied to recognize patterns and classify cellular structures based on their morphological features.
Image Reconstruction
Raw data from laser scanning microscopes often requires processing to produce high-quality images. Deconvolution algorithms remove out-of-focus light, enhancing contrast and resolution.
3D reconstruction techniques combine multiple optical sections to create detailed volumetric representations of samples. This allows researchers to visualize complex structures from any angle.
Super-resolution methods like STED and PALM can break the diffraction limit, achieving resolutions below 100 nm. These techniques require specialized algorithms to process and reconstruct the final images.
Software for Data Processing
Specialized software packages are essential for processing and analyzing laser scanning microscope data. Popular options include ImageJ, Fiji, and CellProfiler, which offer a wide range of analysis tools and plugins.
Commercial software like Imaris and Volocity provide advanced 3D visualization and analysis capabilities. These programs often include user-friendly interfaces for researchers without extensive programming experience.
For more complex analyses, programming languages like Python and MATLAB offer flexibility and customization. Libraries such as scikit-image and OpenCV provide powerful image processing functions for advanced users.
Advancements in Laser Scanning Microscopy
Laser scanning microscopy has undergone significant improvements in recent years. These advancements have expanded the capabilities and applications of this powerful imaging technique across various scientific disciplines.
Super-Resolution Techniques
Laser scanning microscopes now incorporate super-resolution techniques, breaking the diffraction limit of light. This allows researchers to visualize structures at nanometer-scale resolution.
Stimulated emission depletion (STED) microscopy uses two laser beams to achieve resolutions down to 20-30 nanometers. One beam excites fluorophores, while the other depletes fluorescence around the focal point.
Structured illumination microscopy (SIM) employs patterned light to illuminate samples, enhancing resolution by a factor of two. This technique is particularly useful for live-cell imaging due to its lower light intensity requirements.
Single-molecule localization microscopy methods like PALM and STORM enable even higher resolutions by precisely localizing individual fluorescent molecules over multiple imaging cycles.
In Vivo Imaging Improvements
Advancements in laser scanning microscopy have significantly enhanced in vivo imaging capabilities. Multiphoton microscopy allows deeper tissue penetration and reduced phototoxicity.
Adaptive optics systems correct for optical aberrations caused by tissue, improving image quality in thick samples. This technology has been crucial for brain imaging studies.
Novel fluorescent probes and proteins have been developed to enable long-term in vivo imaging with reduced photobleaching and improved signal-to-noise ratios.
Light-sheet microscopy techniques, such as selective plane illumination microscopy (SPIM), provide rapid 3D imaging of large samples with minimal photodamage. This has revolutionized developmental biology studies.
Automation and High-Throughput Systems
Modern laser scanning microscopes feature advanced automation capabilities for high-throughput imaging. Automated stage controls and focus systems enable the scanning of large sample areas or multiple samples in a single experiment.
Machine learning algorithms have been integrated into image acquisition and analysis workflows. These systems can automatically identify regions of interest, optimize imaging parameters, and perform real-time image processing.
Microfluidic devices coupled with laser scanning microscopes allow for automated sample handling and imaging of large numbers of cells or small organisms.
High-speed scanning systems, including resonant scanners and polygon mirror scanners, have dramatically increased image acquisition rates. This enables the capture of rapid biological processes in real-time.
Operational Challenges and Solutions

Laser scanning microscopes face several operational challenges. One major issue is the need for precise movement in the Z direction to find the highest intensity at each pixel. This requires a travel mechanism with nanometer-level resolution at high magnifications.
Another challenge is imaging speed. Traditional laser scanning techniques can be limited by the speed of active beam scanners. FACED (Free-space Angular-Chirp-Enhanced Delay) imaging offers a solution by bypassing active scanners, potentially increasing imaging speed.
Sample preparation presents additional difficulties. Biological samples may be sensitive to light exposure or require specific environmental conditions. Researchers must balance image quality with sample preservation.
Solutions to these challenges include:
- Advanced piezoelectric stages for precise Z-axis control
- Implementation of FACED or other high-speed scanning techniques
- Optimized sample preparation protocols
- Use of long-wavelength multiphoton excitation to reduce photodamage
- Integrated environmental control systems for live cell imaging
Ongoing research focuses on developing more robust, user-friendly systems. Next-generation laser scanning microscopes aim to be turnkey, portable, and suitable for a wide range of applications in both research and industry settings.
Safety and Handling Protocols
Laser scanning microscopes require strict safety measures due to their use of high-powered lasers. Operators must wear appropriate protective eyewear designed for the specific wavelengths used.
Access to laser microscope facilities should be restricted to trained personnel only. Clear signage indicating active laser use must be displayed outside the room.
Proper ventilation is crucial to remove potentially harmful fumes generated during imaging. Regular maintenance checks on ventilation systems are essential.
Equipment should be inspected before each use for any damage or misalignment. Faulty components must be repaired or replaced immediately by qualified technicians.
When not in use, lasers should be powered down completely. Key switches or interlocks can prevent unauthorized activation.
Reflective jewelry and watches should be removed before operating the microscope to avoid inadvertent beam reflections.
Emergency shutdown procedures must be clearly posted and regularly practiced. All users should be familiar with the location of emergency stop buttons.
Samples should be prepared in a separate area to minimize contamination risks. Proper handling and disposal protocols for biological or hazardous materials are critical.
Regular safety training and updates on laser hazards should be provided to all microscope users.
Future Perspectives in Laser Scanning
Laser scanning microscopy continues to evolve rapidly. Advances in technology are paving the way for more powerful and versatile imaging capabilities.
One promising direction is the development of infrared spectroscopic laser scanning confocal microscopy. This technique combines high-resolution imaging with chemical analysis, allowing researchers to visualize and identify specific molecules within samples.
Improvements in speed and resolution are also on the horizon. Light-sheet microscopy is emerging as a fast alternative to traditional laser scanning, enabling rapid 3D imaging of large samples.
Machine learning and artificial intelligence are expected to play a growing role. These technologies can enhance image processing, automate analysis, and extract valuable insights from complex datasets.
Portability is becoming increasingly important. Next-generation multiphoton microscopes are being designed to be more compact and robust, allowing for use outside of traditional laboratory settings.
The integration of multiple imaging modalities into single instruments is another trend. This approach will provide researchers with complementary data from a single sample, offering a more comprehensive view of biological structures and processes.
As these technologies mature, laser scanning microscopy is poised to make significant contributions across various fields, from basic research to clinical applications.