Quantum Metrology: Advancing Precision Measurement Techniques
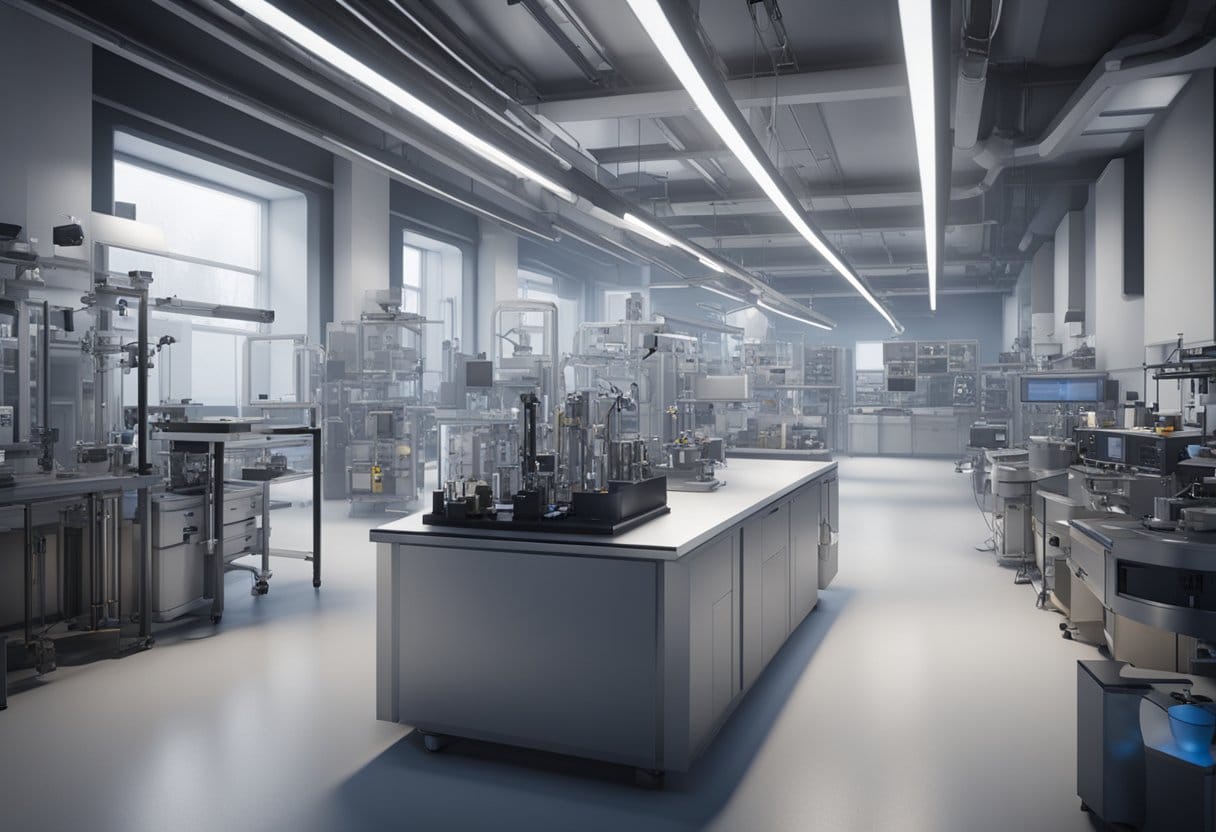
Quantum metrology is a field that uses quantum effects to make super precise measurements.
It's like regular measuring, but with added quantum magic.
Scientists use special quantum states and tricks to get much better results than normal methods allow.

Quantum metrology can measure things more accurately than classical methods, often beating normal limits by a large margin.
This makes it very useful in many areas of science and technology. From improving clocks to detecting tiny magnetic fields, quantum metrology opens up new possibilities.
The ideas behind quantum metrology come from both quantum physics and information theory. By using quantum states like entanglement, scientists can squeeze out extra information from their experiments.
This field is growing fast and may lead to big advances in sensing and measurement technology in the coming years.
Fundamentals of Quantum Metrology
Quantum metrology uses the principles of quantum mechanics to make extremely precise measurements.
It relies on quantum states and effects to improve measurement accuracy beyond classical limits.
Principles of Quantum Mechanics
Quantum mechanics describes the behavior of matter and energy at the smallest scales. Key principles include:
• Superposition: Quantum objects can exist in multiple states at once • Entanglement: Particles can be linked so measuring one affects the other • Wave-particle duality: Matter and energy exhibit both wave and particle properties
These quantum effects allow for unprecedented measurement precision.
Superposition lets quantum sensors detect tiny changes. Entanglement can reduce noise and boost sensitivity.
Quantum systems are very fragile. They easily lose their quantum properties through interaction with the environment. This makes them useful as sensitive detectors.
Quantum Measurement Theory
Quantum measurement theory deals with how we observe quantum systems. Key concepts include:
• Uncertainty principle: Limits on measuring certain pairs of properties • Quantum non-demolition: Measuring without destroying quantum states • Weak measurements: Getting partial info without fully collapsing the state
Coherent quantum states are used to detect physical effects.
The measurement process itself affects the quantum system. This leads to fundamental limits on measurement precision.
Careful measurement design is needed to extract useful information. Quantum error correction helps protect fragile quantum states.
Quantum Enhancements in Metrology
Quantum metrology uses quantum resources to boost measurement accuracy. Common techniques include:
• Squeezed states: Reducing noise in one property at the expense of another • Entangled states: Using quantum correlations to improve sensitivity • Quantum clock transitions: Exploiting energy levels insensitive to disturbances
These allow measurements to surpass classical limits.
Entangled atomic ensembles can measure time and magnetic fields more precisely. Squeezed light improves gravitational wave detection.
Quantum sensing finds uses in navigation, medical imaging, and fundamental physics. As quantum control improves, even more precise measurements become possible.
Key Techniques in Quantum Metrology
Quantum metrology uses advanced methods to make very precise measurements. These techniques allow scientists to measure things more accurately than ever before.
Quantum Entanglement and Sensing
Quantum entanglement is a powerful tool in quantum metrology. It creates links between particles that classical systems can't match. This lets scientists make more precise measurements.
Entangled particles can detect tiny changes in things like magnetic fields or gravity. For example, entangled atoms in an atomic clock can keep time better than non-entangled ones.
Scientists also use quantum squeezing. This technique reduces noise in one property while increasing it in another. It helps measurements go beyond the Standard Quantum Limit, which is a barrier in classical physics.
Quantum Tomography
Quantum tomography is a key method for checking and controlling quantum systems. It's like taking a picture of a quantum state.
Scientists use it to:
- Map out the quantum state of a system
- Check if quantum devices are working right
- Find and fix errors in quantum computers
Tomography involves making many measurements on identical copies of a quantum state. Then, complex math helps rebuild a full picture of the state.
This technique is crucial for making sure quantum sensors and computers work as they should.
Error Correction in Quantum Measuring Systems
Quantum systems are very sensitive to outside noise. This can cause errors in measurements. Error correction helps fix this problem.
Scientists use special codes to protect quantum information. These codes can detect and fix mistakes without disturbing the quantum state.
Some key error correction methods include:
- Surface codes
- Topological codes
- Fault-tolerant quantum computing
These techniques help quantum measurements stay accurate even when there's noise. They're essential for building reliable quantum sensors and computers.
Applications of Quantum Metrology
Quantum metrology enhances measurement accuracy in various fields. It uses quantum properties to achieve better precision than classical methods. This technology has important uses in timekeeping, sensing, and navigation.
Time and Frequency Standards
Quantum metrology improves atomic clocks. These clocks use quantum states of atoms to measure time. They are much more precise than regular clocks.
Atomic clocks based on quantum metrology can be accurate to within one second over billions of years. This level of precision is useful for GPS systems and internet synchronization.
Scientists use trapped ions or neutral atoms in optical lattices for these clocks. The quantum properties of these particles allow for very stable frequency references.
Improved time standards help in finance, telecommunications, and scientific research. They enable better coordination of global networks and more accurate experiments.
Quantum Imaging and Sensing
Quantum metrology enhances imaging and sensing techniques. It allows scientists to see things that were once too small or faint to detect.
Single-photon detection is a key part of quantum sensing. It can measure very weak signals with high accuracy.
Quantum sensors can detect tiny magnetic fields. This is useful in medicine for brain imaging. It also helps in finding minerals underground.
In biology, quantum sensing techniques can study living cells without damaging them. This gives new insights into how cells work and respond to stimuli.
Quantum imaging can create clearer pictures with less light. This is helpful in night vision technology and in looking at distant space objects.
High-Precision Navigation
Quantum metrology improves navigation systems. It makes them more accurate and less reliant on external signals like GPS.
Quantum sensors can detect tiny changes in gravity. This helps create detailed maps of the Earth's surface. These maps are useful for finding resources and predicting natural disasters.
Ultracold atoms are used in quantum navigation devices. They can measure acceleration and rotation very precisely. This is important for aircraft, ships, and submarines.
Quantum navigation systems work even when GPS signals are blocked. This makes them valuable for military and emergency operations. They also help in exploring places where traditional navigation is difficult, like deep underground or in space.
Challenges in Quantum Metrology

Quantum metrology faces several key obstacles that limit its practical implementation and performance. These challenges impact the accuracy and scalability of quantum sensing techniques.
Decoherence and Noise Issues
Decoherence poses a major hurdle in quantum metrology. It causes quantum systems to lose their delicate superposition states. This limits the time available for measurements.
Environmental noise further degrades the precision of quantum sensors. Unwanted interactions with the surroundings introduce errors. These errors can mask the signals scientists aim to detect.
Researchers work on ways to shield quantum systems from noise. They develop error correction methods to extend coherence times. Quantum sensing techniques seek to detect specific physical effects while minimizing unwanted disturbances.
Scalability of Quantum Technologies
Scaling up quantum sensing devices presents technical challenges. Creating large numbers of identical qubits is difficult. Maintaining coherence across many qubits becomes harder as systems grow.
Integrating quantum sensors with classical readout electronics is complex. It requires precise control and measurement at the quantum level. This integration must happen without disrupting the delicate quantum states.
Researchers explore different physical platforms for scalable quantum sensing. These include trapped ions, superconducting circuits, and diamond defects. Each platform has unique strengths and limitations for metrology applications.
Resource Requirements and Optimization
Quantum metrology often demands significant resources. This includes specialized equipment, ultra-cold temperatures, and highly skilled personnel.
Optimizing the use of quantum resources is crucial. Scientists aim to achieve the best measurement precision with limited qubits and time. This involves careful design of quantum states and measurement protocols.
Non-Hermitian quantum metrology explores new ways to improve precision. It seeks to push the limits of measurement accuracy in quantum systems. Researchers investigate how to reach Heisenberg scaling, which offers the ultimate precision allowed by quantum mechanics.
Advancements in Quantum Metrology
Quantum metrology has seen major progress in recent years. New tools and techniques are pushing the boundaries of measurement precision and sensitivity.
Nanotechnology in Quantum Measurements
Quantum metrology now uses tiny nanostructures to get better results.
Scientists create quantum sensors on a very small scale. These nanoscale devices can detect tiny changes in things like magnetic fields or gravity.
One key advance is the use of nitrogen-vacancy centers in diamond. These atomic-scale defects act as super-sensitive quantum probes. They can measure magnetic fields with incredible precision.
Another area of progress is quantum-enhanced atomic force microscopy. This lets researchers see and measure things at the atomic level. It uses quantum effects to get sharper images than normal microscopes.
Quantum Computing's Impact on Metrology
Quantum computers are changing how we do precise measurements. They can process quantum information in new ways. This helps scientists design better experiments and analyze data.
One big step is quantum-enhanced sensing. It uses special quantum states to beat normal measurement limits. For example, squeezed light states can measure gravitational waves more accurately.
Quantum error correction is another key advance. It helps keep quantum sensors working well for longer. This is crucial for things like ultra-precise clocks.
Futuristic Materials for Quantum Sensors
New materials are making quantum sensors even better.
Scientists are finding substances with unique quantum properties. These can be used to build more sensitive detectors.
Topological materials are one exciting area. They have special electronic states that are very stable. This makes them great for quantum sensing. They could lead to sensors that work well even in harsh conditions.
2D materials like graphene are also promising.
They can be made into very thin, flexible quantum sensors. These might be used to detect tiny magnetic fields in living cells.
Researchers are even exploring exotic quantum states of matter.
Things like time crystals could open up new ways to measure time and space.
Integration with Classical Metrology

Quantum metrology and classical metrology are merging to create more powerful measurement techniques.
This combination brings together the best of both worlds, leading to new approaches for improving accuracy and precision in various fields.
Hybrid Quantum-Classical Approaches
Hybrid quantum-classical systems are becoming more common in metrology.
These systems use quantum sensors alongside classical data processing methods.
One example is the use of quantum probes with classical optimization algorithms. This approach helps find the best measurement strategies.
Researchers are also developing quantum-enhanced classical sensors.
These devices use quantum effects to boost the performance of traditional measuring tools.
Standardization and Benchmarking
As quantum metrology advances, there's a growing need for standardization.
This ensures that quantum measurements can be compared across different labs and applications.
Experts are working on creating common benchmarks for quantum sensors. These benchmarks will help assess the performance of new quantum metrology techniques.
Calibration methods are being developed to link quantum measurements to existing classical standards.
This step is crucial for integrating quantum metrology into current measurement systems.
Industrial and Commercial Considerations
Industries are starting to explore the potential of quantum metrology.
Some sectors, like telecommunications and healthcare, are looking at ways to use quantum sensors in their products.
Cost is a major factor in adopting quantum metrology.
Companies are weighing the benefits of improved precision against the expenses of implementing new technologies.
Training programs are being created to prepare workers for using quantum measurement tools. This education is essential for bringing quantum metrology into real-world applications.
Collaborations between research institutions and businesses are helping to bridge the gap between theory and practice.
These partnerships are crucial for turning quantum metrology concepts into useful products.
Outlook and Future Directions
Quantum metrology is poised for major breakthroughs.
New research, policy shifts, and educational initiatives will shape its development in the coming years.
Emerging Research in Quantum Metrology
Scientists are exploring novel quantum systems for enhanced sensing.
Coherent superposition states show promise for detecting subtle physical effects. These may lead to ultra-precise measurements of gravity, magnetic fields, and time.
Quantum entanglement is another active area of study.
Researchers aim to harness entangled particles for improved sensor networks. This could enable distributed sensing over large areas with unprecedented accuracy.
Hybrid quantum-classical systems are gaining attention.
These combine quantum sensors with classical data processing. The goal is to leverage quantum advantages while using familiar computing methods.
Policy and Ethical Aspects
Governments are recognizing quantum metrology's strategic importance.
Many countries are increasing funding for quantum research and development. This includes support for basic science and commercial applications.
Ethical concerns are emerging around quantum sensing capabilities.
There are debates about privacy implications of highly sensitive detectors. Policymakers are working to balance innovation with responsible use.
International cooperation in quantum metrology is growing.
Researchers are calling for open standards and shared resources. This could accelerate progress and ensure global access to quantum technologies.
Educational Implications for Quantum Technologies
Universities are expanding quantum science programs.
New courses cover quantum mechanics, sensing, and computation. These aim to prepare students for careers in quantum technologies.
Industry partnerships are key to quantum education.
Companies are collaborating with schools to develop practical training. This helps bridge the gap between theory and real-world applications.
Online learning platforms are making quantum concepts more accessible.
Free courses and tutorials are available to anyone with internet access. This democratizes knowledge and could spark wider interest in quantum fields.
Hands-on experience with quantum devices is becoming crucial.
Schools are investing in quantum teaching labs. Students can now work directly with quantum sensors and other advanced equipment.