Tensile Testing Machine: Essential Tool for Material Strength Analysis
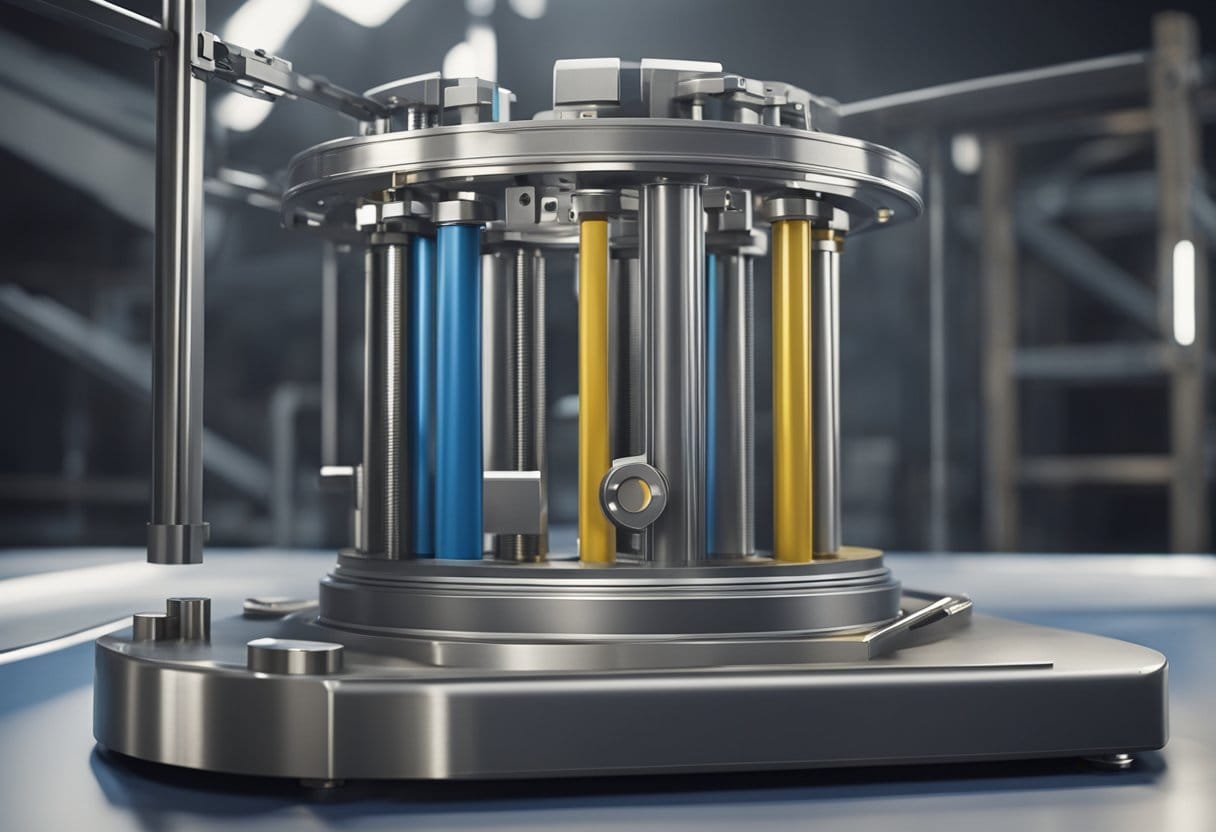
Tensile testing machines are essential tools in materials science and engineering. These sophisticated devices measure the strength and behavior of materials under tension. Universal testing machines can perform tensile, compression, and other mechanical tests on a wide range of materials and products.

Engineers and researchers use tensile testers to evaluate material properties like yield strength, ultimate tensile strength, and elongation. This data is crucial for designing safe and reliable products across industries. From aerospace components to medical devices, tensile testing ensures materials meet required specifications.
Modern tensile testing machines offer advanced features for precise measurements and data analysis. Many systems provide automated testing capabilities and integrate with software for real-time monitoring and reporting. As materials technology evolves, tensile testing remains a fundamental method for quality control and product development.
Basics of Tensile Testing
Tensile testing is a fundamental method for evaluating material properties. It provides crucial data on strength, elasticity, and deformation behavior under uniaxial stress conditions.
Definition and Purpose
A tensile test applies a pulling force to a material specimen until failure occurs. Its primary purpose is to determine how materials respond to applied loads.
Key properties measured include:
- Tensile strength
- Yield strength
- Elastic modulus
- Elongation at break
These tests help engineers select appropriate materials for specific applications. They also aid in quality control and product development processes.
Tensile testing machines typically consist of a load frame, grips to hold the specimen, and sensors to measure force and displacement. The machine gradually increases the applied force while recording the specimen's deformation.
Historical Development
Tensile testing has roots dating back to the Industrial Revolution. Early tests were simple, often using hanging weights to apply loads to materials.
The 19th century saw significant advancements. In 1856, David Kirkaldy built one of the first commercial testing machines in Glasgow. His machine could apply loads up to 1,000,000 pounds (453,592 kg).
By the early 20th century, standardized testing methods emerged. Organizations like ASTM International developed protocols to ensure consistent results across different laboratories.
Modern tensile testers are highly automated. They use sophisticated sensors and computer control systems to provide precise measurements and detailed analysis of material behavior.
Types of Tensile Testing Machines
Tensile testing machines come in several varieties, each with unique capabilities suited for different materials and testing requirements. The main types include electromechanical, hydraulic, and servo-hydraulic machines, which differ in their force generation mechanisms and control systems.
Electromechanical Machines
Electromechanical tensile testing machines use an electric motor to drive a leadscrew, which moves the crosshead. These machines offer precise control and are ideal for lower force applications, typically up to 600 kN. They provide excellent accuracy and repeatability for testing metals, plastics, and composites.
Key features of electromechanical machines include:
- High precision and stability
- Low maintenance requirements
- Quiet operation
- Suitable for both static and limited cyclic testing
Tensile testers in this category often have force capacities ranging from 5 kN to 2,500 kN. They are widely used in quality control and research laboratories due to their versatility and ease of use.
Hydraulic Machines
Hydraulic tensile testing machines utilize hydraulic pressure to generate force. These machines are capable of producing very high forces, making them suitable for testing large specimens or high-strength materials like steel and concrete.
Advantages of hydraulic machines include:
- High force capacity (up to several million newtons)
- Ability to maintain constant load for extended periods
- Robust design for heavy-duty applications
Hydraulic systems can handle large deformations and are often used in construction and heavy industry for testing structural components. They are particularly effective for compression testing of concrete and other building materials.
Servo-Hydraulic Machines
Servo-hydraulic tensile testing machines combine the power of hydraulic systems with the precision of electronic servo controls. This hybrid approach offers the best of both worlds, allowing for high force capacity and accurate control.
Key benefits of servo-hydraulic machines:
- High force capacity with precise control
- Excellent for dynamic and fatigue testing
- Capable of performing complex loading patterns
These machines excel in applications requiring rapid cycling or precise load control. They are commonly used in aerospace, automotive, and advanced materials research where sophisticated testing protocols are necessary. Servo-hydraulic systems can perform a wide range of tests, including tensile, compression, fatigue, and fracture mechanics.
Key Components
Tensile testing machines consist of several essential components that work together to accurately measure material properties. These key elements enable precise force application, measurement, and data collection during testing procedures.
Load Frame
The load frame forms the backbone of a tensile testing machine. It provides structural support and houses other components. Load frames are typically constructed from rigid materials like steel to minimize flexing during testing.
Two common load frame designs are:
- Single column: Compact and suitable for lower force applications
- Dual column: Offers greater stability for higher force tests
The frame incorporates a fixed base and a movable crosshead. The crosshead travels along guide columns, applying tensile force to test specimens. Precision-machined surfaces ensure smooth, aligned motion.
Load capacity is a key specification, ranging from a few newtons to several meganewtons depending on the machine's intended use. Frame stiffness is crucial for accurate results, especially when testing high-strength materials.
Force Measurement System
The force measurement system quantifies the load applied to test specimens. At its core is the load cell, a transducer that converts mechanical force into an electrical signal.
Common load cell types include:
- Strain gauge: Measures minute deformations in a sensing element
- Piezoelectric: Generates electrical charge proportional to applied force
Load cells are calibrated to provide accurate force readings across their specified range. Many machines offer interchangeable load cells to accommodate different test requirements.
Key considerations for force measurement systems:
- Accuracy: Typically 0.5% to 1% of full scale
- Resolution: Ability to detect small force changes
- Range: Matched to expected test loads
Data acquisition systems amplify, filter, and digitize load cell signals for processing and analysis.
Grips and Fixtures
Grips and fixtures securely hold test specimens during testing. Proper selection and use are critical for accurate results.
Common grip types include:
- Wedge grips: Self-tightening for flat or round specimens
- Pneumatic grips: Consistent clamping force for delicate materials
- Hydraulic grips: High-force applications
Grip faces often feature serrated or rubber-coated surfaces to prevent slippage. Custom fixtures accommodate specialized specimen shapes or testing requirements.
Key factors in grip selection:
- Material properties of the test specimen
- Expected test loads
- Temperature conditions
- Specimen geometry
Proper alignment of grips and specimens is essential to avoid introducing bending moments or stress concentrations that could skew results.
Extensometers and Displacement Measurement
Extensometers measure specimen deformation during testing. This data is crucial for determining properties like elastic modulus and yield strength.
Common extensometer types:
- Clip-on: Attached directly to the specimen
- Non-contact: Uses optical or laser technology for measurement
Extensometers offer high precision, typically measuring strains to 0.01% or better. Some designs automatically detach before specimen failure to prevent damage.
For simpler tests, crosshead displacement may be used to estimate strain. However, this method is less accurate due to compliance in the testing system.
Key extensometer specifications:
- Gauge length: Initial measurement span
- Travel: Maximum measurable extension
- Accuracy class: Defined by international standards
Many machines integrate both force and displacement data to generate real-time stress-strain curves during testing.
Control System
The control system coordinates all aspects of machine operation and data collection. Modern tensile testers use computer-based systems with specialized software.
Key control system functions:
- Test parameter setup and execution
- Real-time data acquisition and display
- Safety monitoring and interlocks
- Data analysis and report generation
Control modes typically include:
- Constant crosshead speed
- Constant strain rate
- Load rate control
Advanced systems offer closed-loop feedback, dynamically adjusting test parameters based on real-time measurements. This enables complex test profiles and precise control of loading conditions.
User interfaces range from simple touchscreens to comprehensive software packages. Many systems support customizable test methods, automated specimen handling, and integration with laboratory information systems.
Tensile Testing Procedure
Tensile testing involves a systematic process to accurately measure a material's response to applied forces. The procedure requires careful preparation, precise machine setup, controlled test execution, and thorough data analysis.
Sample Preparation
The first step in tensile testing is preparing the specimen. Materials are machined to standard dimensions according to relevant testing standards. Common specimen shapes include flat bars or cylindrical rods.
Accurate measurements of the sample's cross-sectional area and gauge length are essential. These dimensions directly impact stress and strain calculations. Specimens must be free of defects like scratches or burrs that could affect results.
For some materials, surface treatments or conditioning may be necessary. This could involve polishing metal samples or conditioning polymer specimens to a specific humidity level.
Machine Calibration
Proper calibration of the tensile testing machine ensures accurate results. The load cell, which measures applied force, must be calibrated to known standards. This typically involves using certified weights or a calibration device.
Extensometers or strain gauges used to measure elongation also require calibration. Verification of the crosshead speed is crucial for maintaining the correct strain rate during testing.
Software settings need adjustment to match the specific test parameters. This includes setting appropriate data acquisition rates and defining test end conditions like maximum load or elongation.
Test Execution
To begin the test, the specimen is securely mounted in the machine's grips. Proper alignment is critical to prevent premature failure or inaccurate results. Some tests may require preloading to remove slack.
The tensile test starts by separating the grips at a constant rate, typically measured in inches or millimeters per minute. The rate depends on the material and relevant testing standards.
Throughout the test, the machine records force and elongation data. Many modern systems provide real-time graphing of the stress-strain curve. The test continues until the specimen fractures or reaches a predefined endpoint.
Data Collection and Analysis
As the test progresses, the machine collects force and displacement data at regular intervals. This raw data is then converted into engineering stress and strain values using the specimen's original dimensions.
Key properties determined from the data include:
- Yield strength
- Ultimate tensile strength
- Modulus of elasticity
- Elongation at break
- Reduction in area
Advanced analysis may involve true stress-strain calculations or determination of strain hardening coefficients. Many tensile testing machines come with software that automates these calculations and generates comprehensive test reports.
Visual examination of the fractured specimen can provide additional insights into material behavior and failure modes.
Material Behavior and Test Outcomes
Tensile testing reveals crucial information about a material's mechanical properties. The data obtained provides insights into strength, elasticity, and deformation characteristics under load.
Stress-Strain Curves
Stress-strain curves graphically represent a material's response to applied forces. The x-axis typically shows strain (deformation), while the y-axis displays stress (force per unit area).
These curves illustrate several key material properties:
- Elastic region: Initial linear portion where deformation is reversible
- Yield point: Where plastic deformation begins
- Plastic region: Non-linear section after yielding
- Fracture point: Where material failure occurs
Different materials produce distinct curve shapes. Ductile materials like metals often show a large plastic region, while brittle materials like ceramics have shorter curves with minimal plastic deformation.
Ultimate Tensile Strength
Ultimate tensile strength (UTS) is the maximum stress a material can withstand before fracture. It represents the highest point on the stress-strain curve.
UTS is crucial for:
- Determining material suitability for specific applications
- Comparing strength properties between materials
- Establishing safety factors in design
For example, high-strength steels may have a UTS over 1000 MPa, while some polymers might only reach 50 MPa. Engineers use this data to select appropriate materials for load-bearing structures and components.
Yield Strength
Yield strength marks the transition from elastic to plastic deformation. It's the stress at which a material begins to deform permanently.
Key aspects of yield strength:
- Often defined by a 0.2% offset method for materials without a clear yield point
- Critical for design in applications where permanent deformation is unacceptable
- Generally lower than ultimate tensile strength
Yield strength is particularly important in structural design, ensuring materials operate within their elastic limits under normal conditions.
Elasticity and Plasticity
Elasticity refers to a material's ability to return to its original shape after deformation. Plasticity is the permanent deformation that occurs beyond the elastic limit.
Elastic behavior:
- Characterized by Young's modulus (slope of the elastic region)
- Important for applications requiring dimensional stability
Plastic behavior:
- Indicates ductility or malleability
- Relevant for manufacturing processes like forming and shaping
Materials exhibit varying degrees of elasticity and plasticity. Some, like rubber, have high elasticity but low strength. Others, like certain metals, combine high strength with significant plasticity, making them versatile for many applications.
Testing Standards and Accuracy

Tensile testing machines rely on established standards and precise calibration to produce reliable results. Proper maintenance and adherence to international protocols ensure consistent and accurate measurements across different testing scenarios.
International Testing Standards
ASTM and ISO are the primary organizations that provide global standards for tensile testing. ASTM E8 is a widely used standard for metallic materials, while ISO 6892-1 covers room temperature testing of metallic materials.
These standards define crucial parameters such as:
- Specimen dimensions
- Testing speed
- Data collection methods
- Result interpretation
Adherence to these standards ensures comparability of results between different laboratories and testing facilities. For example, ASTM E8 specifies precise guidelines for specimen preparation and testing procedures for metals.
Factors Affecting Accuracy
Several factors can influence the accuracy of tensile testing results:
- Specimen preparation: Improper machining or handling can introduce stress concentrations.
- Alignment: Misalignment of the specimen in the grips can lead to uneven stress distribution.
- Environmental conditions: Temperature and humidity fluctuations can affect material properties.
- Load cell calibration: Inaccurate load cells can produce false readings.
- Extensometer precision: Poor extensometer calibration can lead to incorrect strain measurements.
To minimize these factors, operators must follow strict protocols and regularly verify equipment calibration. Advanced features in modern tensile testing machines, such as automatic alignment systems and environmental chambers, help mitigate some of these issues.
Role of Machine Maintenance
Regular maintenance is crucial for ensuring the accuracy and reliability of tensile testing machines. Key maintenance tasks include:
- Calibration of load cells and extensometers
- Inspection and cleaning of grips and fixtures
- Lubrication of moving parts
- Software updates and data backup
A well-maintained machine provides more consistent results and reduces the likelihood of unexpected breakdowns. Manufacturers often recommend annual calibration and maintenance schedules to ensure optimal performance.
Proper documentation of maintenance activities and calibration records is essential for quality assurance and compliance with testing standards. This documentation also aids in troubleshooting and identifying long-term performance trends.
Applications and Industries
Tensile testing machines play a crucial role across various sectors, providing essential data on material properties. These versatile devices enable researchers, manufacturers, and engineers to assess and optimize materials for specific applications.
Metallurgy and Materials Science
In metallurgy and materials science, tensile testing machines are indispensable tools for evaluating material properties. Researchers use these devices to determine the strength, ductility, and elasticity of metals, alloys, and composites.
The machines help in developing new materials with enhanced characteristics. They also aid in studying the effects of heat treatment, alloying, and processing methods on material properties.
Data obtained from tensile tests guides material selection for specific applications. This information is vital for designing structures, components, and products that can withstand expected loads and environmental conditions.
Manufacturing Quality Control
Tensile testing machines are extensively used in manufacturing for quality control purposes. They ensure that materials and products meet specified standards and performance requirements.
Industries such as plastics, rubber, textiles, and electronics rely on these machines to verify the consistency and reliability of their products. Regular testing helps identify defects or variations in material properties early in the production process.
Quality control tests using tensile machines can include:
- Yield strength measurement
- Ultimate tensile strength determination
- Elongation at break analysis
- Elastic modulus calculation
These tests help manufacturers maintain product quality, reduce waste, and improve customer satisfaction.
Aerospace and Automotive Testing
The aerospace and automotive industries utilize tensile testing machines to ensure the safety and performance of critical components. These sectors demand materials that can withstand extreme conditions while maintaining structural integrity.
Tensile testing is crucial for evaluating the strength and durability of materials used in vehicle and aircraft components. Engineers test various materials, including metals, composites, and advanced alloys, to optimize performance and weight.
Key applications in these industries include:
- Testing structural materials for aircraft fuselages and wings
- Evaluating automotive body panels and chassis components
- Assessing the performance of lightweight materials for fuel efficiency
- Verifying the strength of safety-critical parts like seat belts and airbag fabrics
Biomedical Applications
In the biomedical field, tensile testing machines are essential for developing and testing materials used in medical devices and implants. These tests ensure the safety and efficacy of products that interact with the human body.
Researchers use tensile testing to evaluate:
- The mechanical properties of artificial tissues and organs
- The strength and flexibility of surgical sutures
- The durability of prosthetic limbs and joint implants
- The elasticity of wound dressings and bandages
Tensile tests also help in understanding the mechanical behavior of natural tissues, aiding in the development of biomimetic materials. This knowledge is crucial for creating medical devices that closely mimic the properties of human tissues and organs.
Innovations and Technological Advancements
Tensile testing machines have undergone significant improvements in recent years. These advancements aim to enhance accuracy, efficiency, and the overall testing process.
One notable innovation is the integration of real-time monitoring capabilities. This allows manufacturers to observe and analyze test samples during the testing procedure, providing valuable insights that were previously unavailable.
Automation has become a key focus in tensile testing technology. Modern machines incorporate advanced automated features, reducing human error and increasing testing speed.
Universal Testing Machines (UTMs) have evolved to handle a wide range of testing requirements. These versatile machines can now accommodate various materials and specimen shapes, expanding their applications across industries.
Data analytics has also played a crucial role in advancing tensile testing. New software solutions enable more comprehensive analysis of test results, leading to better understanding of material properties.
Sustainability is another area of innovation. Manufacturers are developing eco-friendly testing methods and machines that consume less energy and produce minimal waste.
The field of tensile testing continues to evolve, with ongoing research focused on improving precision, efficiency, and adaptability to meet the changing needs of industries relying on material strength testing.
Selection and Purchasing Guidelines

Choosing the right tensile testing machine requires careful consideration of several factors. Capacity is a crucial aspect, as it determines the maximum force the machine can apply. Ensure the selected machine can handle the materials you plan to test.
Accuracy and precision are vital for reliable results. Look for machines with high-resolution load cells and precise displacement measurements. User-friendly software can significantly enhance the testing process, allowing for easy setup and control of parameters.
Consider the range of tests you need to perform. Some machines offer versatility, capable of conducting various mechanical tests beyond tensile testing, such as compression and flexural tests.
Budget is an important factor, but it's essential to balance cost with quality and features. Investing in a reliable machine can save time and resources in the long run.
Key features to evaluate:
- Load capacity
- Speed range
- Data acquisition rate
- Grip options
- Software capabilities
Lastly, consider the manufacturer's reputation and after-sales support. A reputable company can provide valuable assistance in machine setup, maintenance, and troubleshooting.
Safety Measures and Operational Training
Operating a tensile testing machine requires strict adherence to safety protocols and proper training. User-friendly software allows for easy setup, but operators must still exercise caution.
Personal protective equipment (PPE) is essential. This includes safety glasses, gloves, and closed-toe shoes. Long hair should be tied back, and loose clothing avoided to prevent entanglement.
Before testing, operators must inspect the machine for any visible damage or wear. They should check that all safety guards are in place and functioning correctly.
Proper specimen handling is crucial. Operators must securely fasten samples in the machine's jaws to prevent slippage during testing. This helps maintain accuracy and prevents potential injuries.
Training programs should cover:
- Machine operation basics
- Safety features and emergency procedures
- Proper specimen preparation and handling
- Data interpretation and analysis
Regular maintenance checks are vital for safe operation. Tensile testing machines should undergo scheduled inspections to ensure all components are functioning correctly.
Clear signage near the machine should indicate potential hazards and remind operators of safety protocols. This helps reinforce safe practices and reduces the risk of accidents.